1 Introduction: representing science in extended reality
The advent of open databases, research blogs, and live-streamed experiments through advancements in information and communication technologies has significantly broadened the dissemination and accessibility of scientific knowledge to the public. Despite ongoing debates about its roles and values, digital media’s expressive power and diversity continue to shape scientific knowledge. The integration of Extended Reality (XR) and Virtual Reality (VR) into science communication offers promising avenues for making complex topics engaging and understandable to the public. XR, encompassing virtual, augmented, and extended realities, can demystify processes, environments, or concepts that are otherwise challenging to observe. However, the adoption of XR is not without challenges. Beyond the potential for epistemological or cultural biases, XR introduces specific aesthetic and technical hurdles. The absence of proper guidelines could lead to misrepresentation or distortion of scientific facts. Therefore, establishing production and visualization standards is critical. These standards must balance rigidity and flexibility, accommodating the unique needs of scientific disciplines, the complexity of the knowledge communicated, and the diversity of target audiences.
The lack of best practices for XR in science communication creates a significant knowledge gap, leaving scientists and content creators without the guidance needed to deploy these technologies confidently and effectively. Unanswered questions about ensuring factual accuracy, avoiding biases, and considering sensory and cognitive impacts on users highlight the need for adaptable standards. These standards must evolve with rapid technological advancements and respect the nuances of scientific disciplines and audience needs.
Drawing from a rich, multidisciplinary base encompassing computer graphics, narratology [Rubio-Tamayo, Barrio & García, 2017], human-computer interaction, cognitive science [Markowitz & Bailenson, 2019], and communication studies, this paper outlines a framework for the use of XR as a system for scientific communication. The idea of VR as “systems” was first postulated by Biocca and Levy [1995] and was later taken up by other authors such as Marini, Folgieri, Gadia and Rizzi [2012] who proposed VR as a communication process between humans and information, mediated by computers, and involving factors such as visualization and sensory stimuli and interaction. Houck, Hassan, Thiis and Solheim [2013] further underlined VR’s role as a set of pivotal communication tools that bridge diverse disciplines through interactions with virtual objects, images, and spaces. In addition to these foundational studies, this paper synthesizes technical systems with cognitive phenomena like the “chameleon effect” [Bailenson & Yee, 2005] and the uncanny valley effect, originally identified by Mori [1970, 2012]. Linking systems-theory with perception studies helps to underscore the complexity of social construction within XR environments.
In education and academia, XR and VR have revolutionized the way we simulate experiments and environments, facilitating multisensory learning and interactive study tools across various disciplines. The concept of using virtual reality in education, including extended reality, was pioneered over three decades ago by visionaries like Helsel [1992] and Wickens [1992]. Contemporary research, such as that by Azevich [2019], delves into XR and VR’s pedagogical benefits, underscoring their transformative potential. Insights from fields as diverse as 3D computer graphics, archaeology, STEM, and communication sciences enrich our understanding and application of these technologies in science communication.
The development of XR and VR standards in science communication is pivotal for enhancing the dissemination and impact of scientific knowledge. Such standards should ensure that the representation of scientific results in XR and VR closely aligns with the original research findings, maintaining a high fidelity to the studied facts or phenomena. This foundational principle is crucial for the integrity of science communication. Moreover, the clarity and immersive nature of XR and VR offer additional advantages, such as highlighting unexplored areas within scientific knowledge, thereby guiding future research directions.
Developing standards for XR and VR in science communication can help scientists disseminate results and increase the impact of scientific knowledge that can, ideally, solve society’s most pressing problems. First and foremost, the content of scientific communication must have a maximum degree of correspondence with the fact or phenomenon studied in the research. This correspondence between scientific results and the XR or VR content must be investigated as an elementary factor in the discipline itself. However, the use of XR and VR could have secondary benefits as clearer dissemination through these mediums could contribute to identifying gaps that scientific knowledge itself has not yet reached.
2 Research questions and objectives
This research builds upon existing frameworks for cultural heritage representation and information digitization, aiming to refine and expand these models for the XR/VR domain. Our investigation is steered by two principal research questions:
-
How can fundamental features of communication like narrative and storytelling be applied to science communication in XR/VR?
-
What standards are necessary to ensure the accurate depiction of scientific research findings in XR/VR environments, given the complexities of science communication?
To answer these questions, the study aims to adapt and connect features from existing communication and representation models, tailoring them for the dissemination of scientific knowledge via the immersive and interactive capabilities of XR/VR.
The main objectives are:
-
Analyze augmented reality communication and representation characteristics, including virtual and augmented reality.
-
Conceptualize how to represent different scientific ideas/objects with XR/VR media and determine the most accurate representation approaches based on established visual correspondence parameters.
-
Adapt prior representation theories and standards from different knowledge domains to a 3D scientific knowledge representation model for virtual experiences by describing immersive VR/XR scenario elements.
Through these focused objectives, guided by our central research questions, the study seeks to connect and extend existing models of representation into a comprehensive framework for the effective and standardized communication of scientific knowledge using the evolving technologies of XR and VR.
3 Methodological and research framework
Our research is anchored in the foundational frameworks provided by the London Charter and the Seville Principles, which have set the standards for 3D representations in disciplines such as archaeology and cultural heritage. These disciplines, which fall under the broader umbrella of the humanities and historical research, have benefited from these guidelines in documenting and analyzing historical and archaeological sites. The guidelines address various critical aspects, including the specificity of the represented environment, the accuracy of the portrayed information, ethical considerations, user interaction, and the fidelity of the representation to the original data. Applying these standards to cultural heritage has proven invaluable, offering a robust framework for baselines in the depiction of scientific knowledge. This is particularly relevant in the context of XR and VR, where the challenge lies in accurately representing complex scientific concepts.
To adapt and extend these principles to science communication in XR and VR, we conducted a narrative literature review focusing on scientific communication and knowledge representation using XR and VR. This review aims to identify critical features from various disciplines and how they might inform a model for XR/VR in science communication. This step also involves a critical assessment of the current research landscape to pinpoint gaps and explore potential new applications of XR/VR in scientific contexts.
Following the literature review, our research proceeds with a content analysis of the London Charter and the Seville Principles to extrapolate the main features and standards of these guidelines that could be applied to the representation of scientific knowledge in XR and VR. Our analysis of these texts helps us draw parallels between the established practices in cultural heritage representation and the emerging needs of science communication. This comparative analysis is pivotal in crafting a model that harnesses XR/VR’s immersive and interactive capabilities to make scientific communication engaging and precise.
The analysis also establishes the criteria that define the units of representation and their levels of representation. Two taxonomical classifications of information provide further support for immersive and interactive digital media such as XR/VR. First, the degree of iconicity discussed by Gallego [1985] focuses on the levels of abstraction from a semiotic approach and deals with the relationship of resemblance or similarity between the two aspects of a sign, its meaning, and its forms. Degrees of iconicity may range from the real object in its natural form to a nonfigurative representation of this object. Second, the scales of evidence of Aparicio Resco and Figueiredo [2014] address the accuracy of historical/archeological evidence for its representation in virtual environments. Both the degrees of iconicity and scales of evidence models are helpful for the development of guidelines and help keep in mind variables such as representation, interaction, and immersion, among others.
Our framework identifies key variables that should evolve alongside advancements in augmented reality technology. These include the degree of iconicity, which gauges the visual similarity between represented and actual elements, and the complexity of the information represented in XR and VR media. This development process aims to provide a benchmark for evaluating the viability of depicting scientific phenomena through interactive elements and narrative threads, acknowledging augmented reality’s expressive capacity and the specifics of scientific knowledge to establish representation criteria.
Finally, we conduct a comparative analysis of XR and VR’s main features, characteristics, and underlying technologies. The foundational aspects of the London Charter and Seville Principles serve as a conceptual springboard for devising comprehensive new models. As VR and XR continue to evolve as complex media, it is crucial to adapt and integrate pre-existing domain model features. The proposed framework endeavors to facilitate the scientific representation of phenomena in augmented reality, enabling interactive elements to span various representational levels. This systematic approach aims not only to bridge historical practices with modern technology but also to innovate in the science communication field through XR and VR.
4 Literature review
4.1 Virtual and Extended Reality as media and technical features
XR and VR technologies are evolving at a rapid pace, leading to continual advancements in their narrative and rhetorical capabilities. Despite these changes, two key features consistently underline their potential: the ability to simulate physical environments and facilitate user interaction within these virtual spaces. These capabilities enable XR and VR to offer powerful expressive and representational opportunities. They allow for the representation of text, 3D objects, images, visual effects, and sounds, providing users with a compelling sense of presence within the generated environments. This makes XR and VR invaluable tools for developing guidelines in scientific communication, where the accurate and immersive portrayal of information is crucial.
Utilizing digital information, XR and VR can emulate the experience of being in a real or fictional environment, enhancing the sense of immersion even in fantastical settings. This is achieved through the integration of 3D objects with varying levels of interactivity, offering users the freedom to look around (three degrees of freedom, or 3doF) or move within the environment (six degrees of freedom, or 6doF). Such capabilities are essential for creating engaging and interactive scientific communication experiences.
Despite their powerful expressive potential, academic exploration of XR and VR in science communication remains limited. Tham et al. [2018] highlighted the need for a deeper understanding of VR elements like presence and embodiment to enhance the accuracy and efficiency of fact communication. Similarly, Wuebben, Rubio-Tamayo, Gertrudix Barrio and Romero-Luis [2023] focused on science communication through 360 videos, outlining specific production and narrative considerations that distinguish 360 video from traditional 2D formats. These studies underscore the importance of technology in accurately conveying scientific facts and narratives, pointing to the gap between XR/VR’s potential and its current utilization in academic research on scientific communication.
Other studies identify the components that form part of the VR experience and configure the narrative. Taborda-Hernández, Rubio-Tamayo and Rajas Fernández [2022] focus on the “components of significance of the story in VR”, which includes the combination of the virtual landscapes (VL), the virtual atrezzo (VA), plot key objects (PKO), plot key actions (PKA), parallel stories (PS) and main plot (MP). Those components are explained in Table 1 and illustrated in Figure 1.
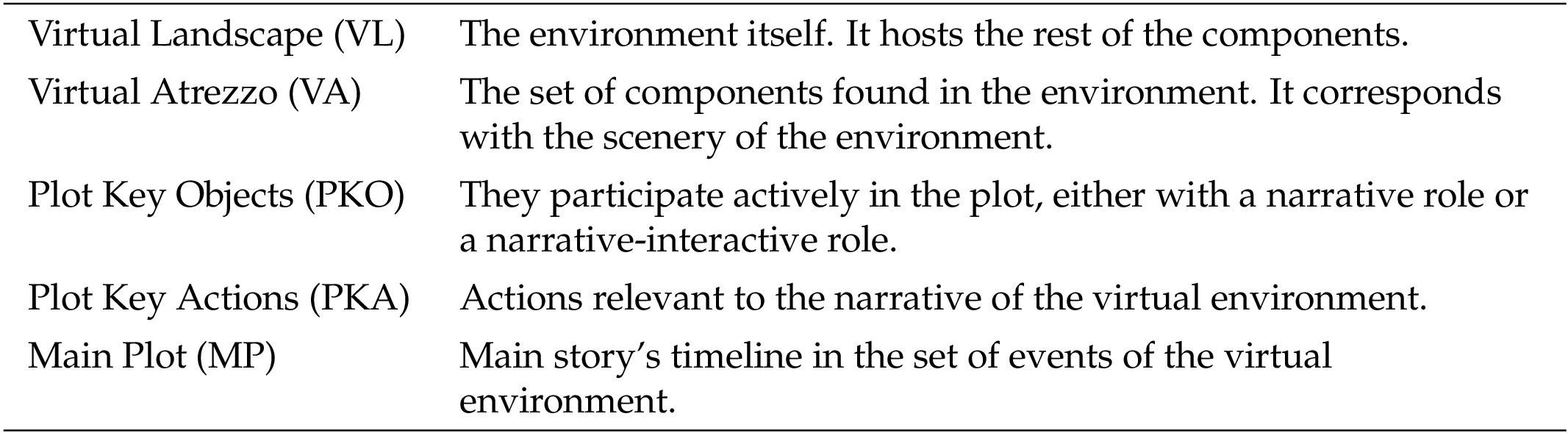
The identification of these components determines the importance of an element’s structure in the plot. In scientific communication, this is crucial for evolving the design of guidelines. These guidelines establish standards for creating immersive and interactive experiences in virtual reality. They also aim to transmit scientific knowledge effectively to various types of users.
4.2 The narrative factor of XR and VR
Narrative plays a crucial role in media evolution, particularly in immersive technologies like XR and VR, which offer interactive experiences with nearly limitless narrative possibilities. These technologies enhance science communication by enabling “transversal content” creation, where users engage with content integrated within a structured, virtual environment. The design process aims to impart knowledge through a narrative shaped by the informative goals of the experience, emphasizing the necessity of narrative in representing and disseminating information effectively.
Narrative and storytelling are pivotal in science communication, serving as the foundations for generating “transversal content” that enhances the representation of scientific concepts with a focus on accuracy, traceability, and adherence to the scientific method. In the realm of XR, the narrative potential is significantly amplified, where every element — whether text, photographs, or immersive 3D objects — plays a critical role in constructing the story. This complexity underscores the assertion that no medium exists without narrative — every form of media inherently encapsulates a narrative, shaping the discourse and composition of the story, especially in contexts where the clarity and integrity of scientific communication are paramount. Thus, developing a narrative approach that not only aligns with but also amplifies these principles is essential for the effective dissemination of scientific knowledge, ensuring that even the most basic immersive experiences contribute meaningfully to the broader discourse of science.
The evolution of narrative theory in virtual reality is supported by scholars like Ryan [2003] and Aylett and Louchart [2003], with contributions from a range of studies exploring immersive narratives and their applications in XR. This body of work forms the basis for developing an Immersive Communication model in extended reality, highlighting the importance of narrative structure in the medium. As the medium has evolved, scholars such as Liestøl [2011], Ryan [2015], Bucher [2017], Mills, Courtney, Dede, Dressen and Gant [2020], Weech, Kenny, Lenizky and Barnett-Cowan [2020], Dincelli and Yayla [2022], and Vallance and Towndrow [2022] have elaborated a range of narrative theories related to XR. Notably, studies like Harley, Tarun, Germinario and Mazalek [2017] delve into the diegesis of 3D objects within immersive narratives in virtual reality, which significantly contributes to the development of an Immersive Communication model in extended reality (XR).
The importance of narrative and storytelling in science communication has been underscored by various authors, highlighting its role in effectively conveying complex and abstract scientific concepts. Joubert, Davis and Metcalfe [2019] emphasize storytelling’s significant potential in science communication, exploring diverse methodologies within the research landscape. Similarly, Martinez-Conde and Macknik [2017] address the criticality of narrative techniques in enhancing the efficiency of science communication, pointing out the complexities and interdependencies of various contributing factors. Further, Green, Grorud-Colvert and Mannix [2018] propose a structured process for embedding storytelling into science communication, which rests on six foundational pillars: learning, implementation, practice, telling, discussion, and reflection. Suzuki, Feliú-Mójer, Hasson, Yehuda and Zarate [2018] delve into the role of storytelling in formulating and communicating scientific concepts, particularly those that are complex, abstract, and challenging to represent, with a focus on cognitive communication strategies.
In addition to narrative aspects, Finkler and Leon [2019] have developed a conceptual framework tailored for the audio-visual format in science communication, highlighting the significance of rhetorical and visual language in content creation. This discussion extends to the representation models, including Villafañe’s Iconicity Scale [Gallego, 1985] and the Scale of Historical Evidence [Aparicio Resco & Figueiredo, 2014]. These models provide a theoretical basis for the relationship between an item’s original form and its representation across different mediums, offering valuable insights into the visual and rhetorical aspects crucial for effective science communication.
There are notable examples of virtual and extended reality applications across various research domains within science communication. Barnidge et al. [2022] explore immersive journalism for disseminating climate science knowledge. Similarly, Tibaldi et al. [2020] apply VR in vulcanology research, also emphasizing its utility in science communication and education. Helbig et al. [2014] delve into 3D visualization of atmospheric data in VR environments, showcasing the potential for immersive information representation. Simões, Morais and Moreira [2019] investigate the integration of multimedia and VR in science centers, focusing on how these technologies aid in communicating research achievements to the public. Or, finally, approaching VR as a tool for research, dissemination and mediation in the field of humanities, through projects such as VESPACE [François, Leichman, Laroche & Rubellin, 2021].
4.3 XR and VR in science education and an approach to science communication
In the realm of science communication, the integration of XR and VR with science education has garnered significant attention, evidenced by studies from Jackson, Taylor and Winn [1999], Allison and Hodges [2000], Shin [2002], Piovesan, Passerino and Pereira [2012], Johnston, Olivas, Steele, Smith and Bailey [2018], Durukan, Artun and Temur [2020] and Matovu et al. [2023]. The expertise developed in crafting science-themed virtual reality content for educational purposes highlights the potential to bridge the gap between education and communication [Riva, 1999]. VR, as a hybrid medium, necessitates that the design of interactions across various components — still images, videos, 3D, and sound — be intricately linked to an overarching narrative and facilitate interaction with new knowledge and environments.
Experimental XR and VR environments, like the early ScienceSpace project by Dede, Salzman and Loftin [1996], showcased the potential of virtual environments in enhancing the comprehension of scientific knowledge. These initial endeavors contributed to the development of standards such as the London Charter. The rapid evolution of video technologies and web tools has further accelerated the application of XR and VR in science education, making the representation of complex concepts more accessible in fields like astronomy [Mintz, Litvak & Yair, 2001] and biology [Shim et al., 2003].
The use of VRML for engineering studies [Manseur, 2005] and the development of motion systems within virtual environments [Chen, Yang, Shen & Jeng, 2007] highlight the expansion of narrative-driven educational experiences in sciences. Systematic reviews by Freina and Ott [2015] and Kavanagh, Luxton-Reilly, Wuensche and Plimmer [2017] have dissected the diverse applications of virtual reality in education, identifying key factors like gamification and constructivist pedagogical methodologies that enhance classroom experiences.
Comparative studies, such as those by Lamb and Etopio [2019] and Liou and Chang [2018], have evaluated the effectiveness of XR and VR as supplements to traditional learning processes, emphasizing their role in boosting motivation and content assimilation. Durukan et al. [2020] and Makransky, Petersen and Klingenberg [2020] each discuss the evolving language of XR and VR and their impact on student retention of knowledge, suggesting immersive technology’s potential for providing effective feedback processes.
The application of XR and VR technologies is increasingly common in STEM education, as highlighted by Al-Azawi, Albadi, Moghaddas and Westlake [2019], reflecting a trend towards integrating technological advancements with scientific education. Research by Matovu et al. [2023] emphasizes the need for a systematic design, implementation, and evaluation process for VR in science learning, highlighting the importance of establishing standards for scientific communication within XR and VR. Such standards ensure fidelity to scientific data and optimize representation in immersive mediums. The development of dedicated disciplines for scientific communication in XR and VR involves an interdisciplinary approach, incorporating fields like interaction design, storytelling, and human-computer interaction. Establishing guiding principles, akin to the Seville Principles or the London Charter, is crucial for standardizing content development in immersive learning environments, underscoring the boundless potential for representing scientific phenomena in extended reality.
4.4 The London Charter and the Seville Principles in heritage
The use of three-dimensional digital media for communication and visualizing content has been applied in various fields, most notably in history and archaeology. However, certain standards and norms needed to be established to ensure a meaningful connection between representation and reality. Considering that 3D objects can be depicted with varying levels of abstraction and symbolism, the ideal level of representation that maximizes efficiency and accuracy must be examined. Despite different levels and types of abstraction and synthesis, the information must faithfully capture the essence of the phenomenon being represented.
The London Charter and Seville Principles serve as widely accepted and influential guidelines for utilizing a particular technology in a specific field of knowledge. They are developed in the first two decades of the 21st century with the purpose of representing cultural heritage through computer graphics and developing standards and guidelines for this aim. Even if both models have different approaches, which are further explained in the adaptation of this model, the contribution of both models is essential for a scientific and rigorous representation of a domain of knowledge and discipline such as, in this case, cultural heritage. They focus also on the term “virtual archaeology”, by highlighting the idea that the information can be represented by the means of computer graphics and standardized. They are one of the first approaches to standardizing the visualization of information with the help of 2D and 3D computer graphics in a scientific discipline and with the aims of preservation and dissemination.
The London Charter [2006; Denard, 2012] for the computer visualization of cultural heritage is created with the aim of establishing a set of principles and guidelines in the representation of archaeological items in a virtual version. It was conceived to develop a set of principles “technically rigorous as longer established cultural heritage research and communication methods” [Denard, 2012, Preamble and Objectives]. It has six principles, based on six pillars to be considered, as follows: Implementation, Aims and Methods, Research Sources, Documentation, Sustainability, and Access. The primary text may be accessed on the following domain [https://londoncharter.org/].
The Seville Principles [2017] are international principles of virtual archeology which offer a list of objectives related to virtual representation of heritage. While the subject of study and representation is similar to the London Charter, the Seville Principles offer distinct approaches and establish definitions for related terms, such as virtual archaeology, archaeological heritage, virtual reconstruction, and virtual recreation, among others. The eight principles of Seville include: Interdisciplinarity, Purpose, Complementarity, Authenticity, Historical Rigour, Efficiency, Scientific Transparency, and Training and Evaluation [The Seville Principles, 2017].
Thus, both the London Charter and the Seville Principles are focused on archaeology but consider contributions of other disciplines and the relevance of digital media. It’s important to note that each media technology and system has its own unique characteristics and areas of expertise. Scholars like Denard [2013] emphasize this point, acknowledging that both the technology itself (such as 3D representation systems) and the specific fields of knowledge in which it is applied continually evolve over time.
The London Charter, rooted in archaeological standards, extends its application beyond its original disciplinary boundaries, raising questions about its relevance. In the realm of information representation across 2D, 3D, and extended reality technologies, diverse approaches incorporating insights from various disciplines emerge as direct derivatives. For instance, principles governing the digital representation of scientific knowledge may differ between science communication and science education. Academic literature emphasizes the significant influence of the intersection between science and representation, with a predominant focus on educational applications. Clear principles for communication are yet to be fully developed, necessitating an open, transparent, inter-disciplinary, and intra-disciplinary process. Emphasis should be placed on applying these principles to create communicative and informative scientific content, thereby influencing the development of educational materials.
Representation in virtual space and with 2D and 3D technologies presents a practically unlimited degree of possibilities that are only going to evolve in the coming years. Through disciplines such as ergonomics, user interaction, and design, we can understand how people interact with the physical environment. Some of this knowledge has been transferred to the design of extended realities. In addition, the limitations of the devices themselves, the inputs-outputs of the users on the space, or the limitations in the correspondence between the virtual space designed and the physical space that can be traversed must be considered. These factors impact the technological transition to more complex technologies and yet, developing common standards can help optimize and efficiently carry out this communication, maintaining the principle of equivalence between the scientific phenomena — data and experiences cultivated through scientific methods — and the represented knowledge, including its narrative structures. Creating such a framework can help promote content that is more comprehensible for the user and generates a greater degree of interest.
5 A framework for science representation and communication in XR and VR
5.1 Features of XR and VR for represent information
Based on this literature review and identification of the main features of different models and approaches, such as those mentioned, the authors of this research have developed a framework with the aim to be applied to the research area of science communication, by considering, science representation and application of extended and virtual reality on science education. The number of models and the complexity of the medium of XR/VR makes it a puzzle with pieces that are difficult to fit together, but necessary.
The standards for science communication in XR and VR can be subdivided into several categories. Therefore, the following are General Operating Principles related to technology and its adaptation to the story, and then the principles that would be an adaptation of those principles of the London Charter and the Seville Principles that may have an equivalent in these new principles.
In the following, then, we detail the general principles of how virtual reality works and its implication in the field of scientific communication. The overall principles (general operating principles) approaching the virtual and extended reality in the field of scientific communication, identified by the authors of this manuscript from the literature review in applications of XR/VR, are the following ones:
-
Virtual reality makes it possible to represent, in immersive first-person virtual environments, information of different kinds: 2D images, 3D objects, video, special sound, etc., and other sensory inputs we receive in the physical world. It has the potential to reconstruct and recombine different kinds of visual, auditory, and tactile sensations. These combinations allow for scientific communication to incorporate the highest possible degree of fidelity.
-
This factor also allows for two types of potential environments to be generated: a faithful representation or more symbolic reconstruction. In either case, the elements represented must have an informative or didactic function revealing an attempt to produce new information. For example, data visualizations and graphics of different colors, sizes, and measurements may be peripheral functions for the understanding of the main story. The symbols appear as if part of a real, physical space. The equivalence is applicable in an analogous way to an artificial environment designed to be visualized in virtual reality.
-
The levels of symbolic representation and abstraction may differ. However, they must always represent the object as faithfully as possible. Any modifications must be clearly and concisely indicated. The most immediate model is the equivalence of symbolic representation in other media in which this representation is fully understood, considering that virtuality adds the variables of potential interaction and increased viewpoints through three-dimensionality in the displacement in space.
-
The representation also must follow the postulates of the scientific method, insofar as it cannot provide information that is incorrect or misleading. Likewise, elements must be incorporated to integrate the traceability of the original source, be it the scientific publication, the data, etc., into the environment, so that all information is traceable and verifiable.
-
If a phenomenon has been extensively studied, references and citations should always give credit to the existing scientific literature.
-
A usability study and the involvement of multidisciplinary profiles are necessary to optimize the representation with respect to the phenomenon represented.
-
If there is a fictitious, symbolic, or abstract element to represent a specific scientific phenomenon, it should be clearly and concisely indicated.
-
Rhetorical figures, insofar as natural language is a complex construct, can contribute to the representation of a particular phenomenon, especially if its literal visual representation is complex. It is necessary to make explicit such phenomena and why they have been represented in this way, using rhetorical figures.
-
Many scientific concepts require an enormous degree of abstraction. This is the case, for example, with quantum physics. In this case, strategies must be devised that optimize the potential representation of the complexity of the phenomena studied in a way that is as equivalent as possible to the idea to be represented and, at the same time, as comprehensible as possible. Develop a science of representation of scientific phenomena, based on STEM disciplines
-
There are several different levels of narrative. On the one hand, the narrative of the scientific fact itself must be verifiable and traceable through reference to the source of the research conducted. Parallel narratives can be developed that serve the primary narrative, if they contribute to a greater understanding of the phenomenon and that they make clear the difference between the main narrative (the fact) and peripheral stories and contexts (narrative additions to the understanding of the phenomenon). On the other hand, the model proposed in the current publication incorporates an adaptation of the principles developed in the London Charter, especially those which call for a faithful “correspondence” between the concept represented and the medium of representation, the former being the scientific information and the latter the medium. This implies that the accuracy of the narrative should be considered in relation to the virtual technologies used to represent it.
All these factors are relevant to understanding the medium of virtual and extended reality, and how other procedures related to computer graphics work. For sketching a framework set in front of scientific communication and extended and virtual reality technologies, it is necessary to understand how computer graphics and virtual reality work and put on the table research related to this domain, as well as pre-existent frameworks.
5.2 London Charter: principles and adaptation to the proposed model
The authors’ proposed model for XR/VR for science representation and communication, is, as explained, a framework that helps to understand how to design and structure information in immersive environments with some level of interaction, such as virtual and extended reality. As shown in Table 2, a comparative focus with the London Charter has been drawn to better understand the model proposed. The differences include, on the one hand, the origin of the information and, on the other hand, the medium in which the information is represented. In addition, as the London Charter was published in 2006, technologies have advanced dramatically in the intervening decades allowing for new possibilities and requiring certain adaptations.

Thus, as the framework proposed is a model based on (in part) the London Charter, the principles have been also considered to be adapted. This adaptation of these principles is determined by the disciplinary values of archaeology, a multifactorial field of knowledge in which representation is scaled. But, in fact, the new model proposed must be adapted to science communication.
For example, an adaptation to the XR/VR Model of Science Representation and Communication, based on London Charter Principle 2 “Aims and Methods” should be carried out as follows:
-
Virtual reality is not the medium of representation par excellence, but it is a complete and complex medium of representation. Knowledge can be understood through other more traditional media, such as written text or images. In fact, for the moment, the scientific community highly values the publication of scientific findings in peer-reviewed academic journals, although their dissemination is often feasible by other means such as social media, 360 videos, and XR.
In the case of Principle 3, “Research Sources”, would be adapted from the London Charter as follows:
-
The scientific knowledge process reveals various sources of research. The process and results are independent of their subsequent dissemination, although their representation in media such as virtual reality can contribute to the illustration of the concepts presented therein and their dissemination. Therefore, the representation should be contextualized by the research surrounding the scientific finding (cited research, established methods) and its research environment (university, government, or private laboratory).
The next table (Table 3) explains how the principles of the London Charter have been adapted to science representation and communication through the analogy between the representation of information between disciplines.
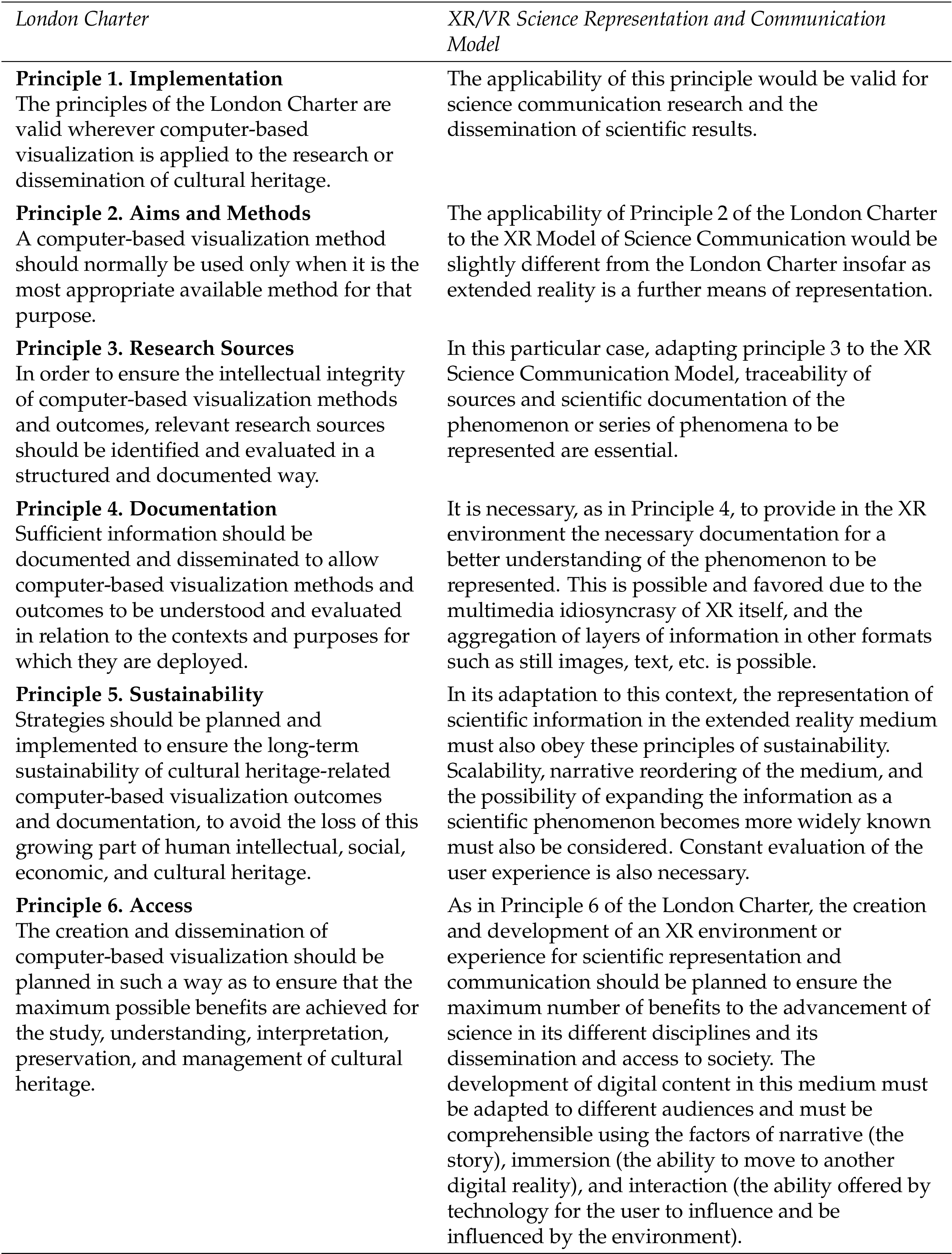
5.3 Adaptations of the Seville Principles
The six Seville Principles [2017], established as guidelines for computer-based visualizations in archaeology and heritage, seek to refine and standardize concepts such as virtual archaeology, management, and virtual reconstruction. Developed after the London Charter [2006], these principles aim to make criteria understandable and applicable, promoting responsible digital technology use. While they share some goals and approaches with the London Charter, the Seville Principles are distinct, often serving complementary purposes. The ensemble of eight principles provides a comprehensive framework detailed in Table 4.
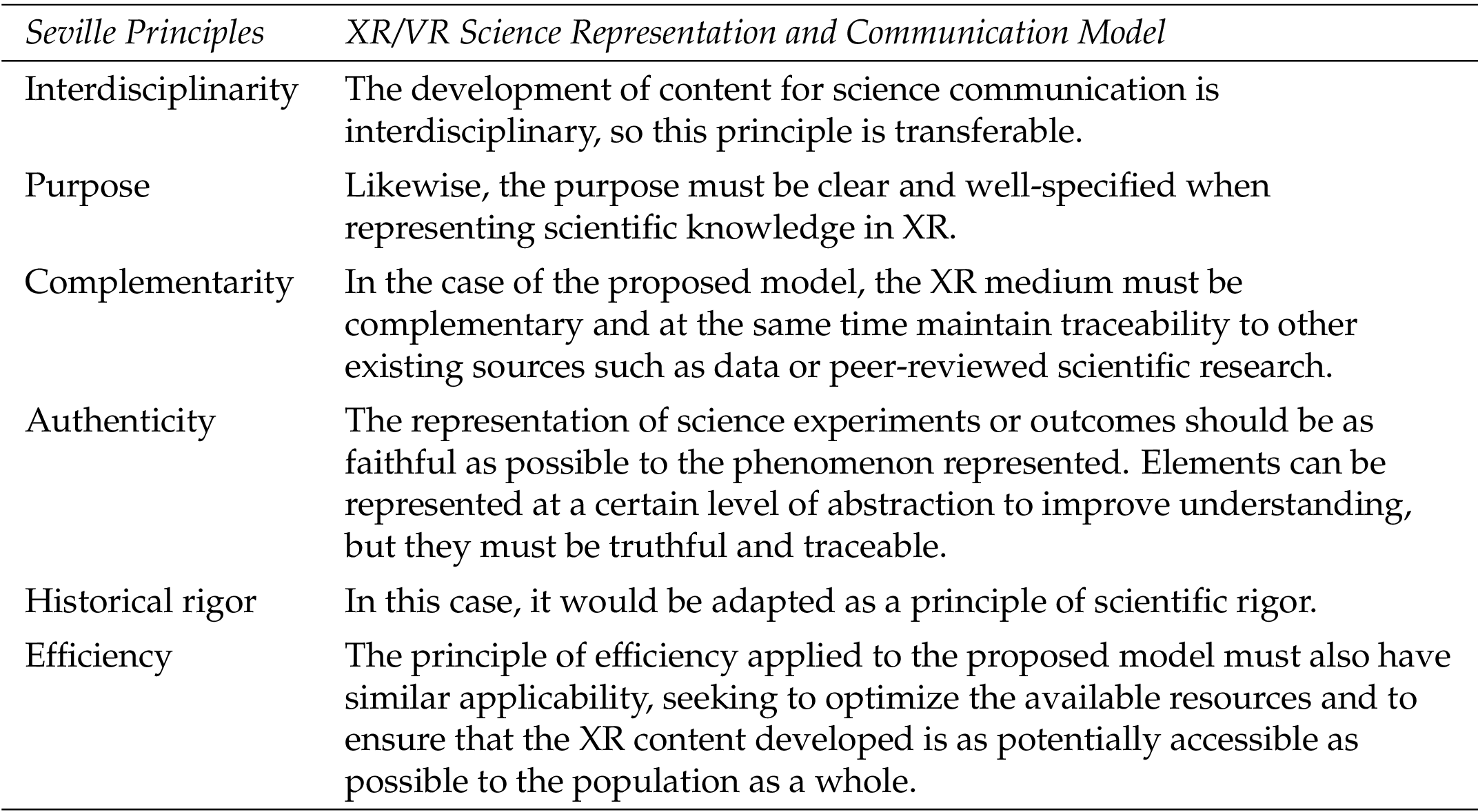
These guidelines recognize the unique challenges and features of scientific representation, advocating for interdisciplinary approaches to optimize visualization in extended reality (XR) environments. The Seville Principles [2017] specifically address the nexus between scientific methodology and the knowledge gleaned from representing environments in immersive and non-immersive virtual media.
The document below outlines how the Seville Principles could be adapted to the XR Science Representation and Communication Model. This adaptation involves reinterpreting the principles for a context where science is represented, considering XR/VR’s interactive and immersive qualities. Unlike the original focus of the Seville Principles (and the London Charter) on computer graphics, this adaptation considers the broader spectrum of digital representation within XR/VR environments, showcasing the potential for integrating these guidelines into scientific communication.
5.4 Adaptation of other levels of representation and degrees of rhetorical matching
Both the London Charter and Seville Principles were developed for archaeology, which often sets its focus on cultures or objects engaged with by humans. In archaeology, historical evidence is represented by categorizing the relationship between the represented object and the physical remains of the actual object. This can be observed in the Scale of Historical Evidence developed by Aparicio Resco and Figueiredo [2014] (Figure 2),
which is influenced by the Bizantium 1200 project. Furthermore, this scale has been enhanced through various approaches, including the subsequent studies by Cáceres-Criado, García-Molina, Mesas-Carrascosa and Triviño-Tarradas [2023] (Figure 3). Therefore, the XR or VR media is reconstructed from a physical object or space.
Yet for many scientific disciplines, the “represented object” does not have a realistic visual equivalence to the represented concept. For example, we cannot represent radio waves with a standard camera, and some concepts, such as bird migration flows, cannot be readily captured even with documentary evidence of birds or flocks in flight. The point is that XR as a medium can potentially accommodate all these representational models, although it is necessary to analyze the nature of the medium and determine the representational need.
The Scale of Historical Evidence [Aparicio Resco & Figueiredo, 2014] and similar models proposed by Cáceres-Criado et al. [2023] employ a color scale to gauge the correspondence between an item’s original and represented forms. This color-coding aids in depicting items in documents, plans, and reconstructions, emphasizing digital media applications. Such scales are invaluable for identifying items in representations and can be applied to various taxonomies and models for clarity and consistency.
Exploring the degree of correspondence between original and represented forms allows for applying these concepts to scientific dissemination. In archaeology, the spectrum from “imagination” to “existence” highlights the importance of representability, tracing the journey from abstract concepts to precise 3D replicas with high resolution. Details of this progression are further outlined in Table 5, contextualized within representation frameworks, and acknowledging the pre-existing evidence criteria in various scientific domains.
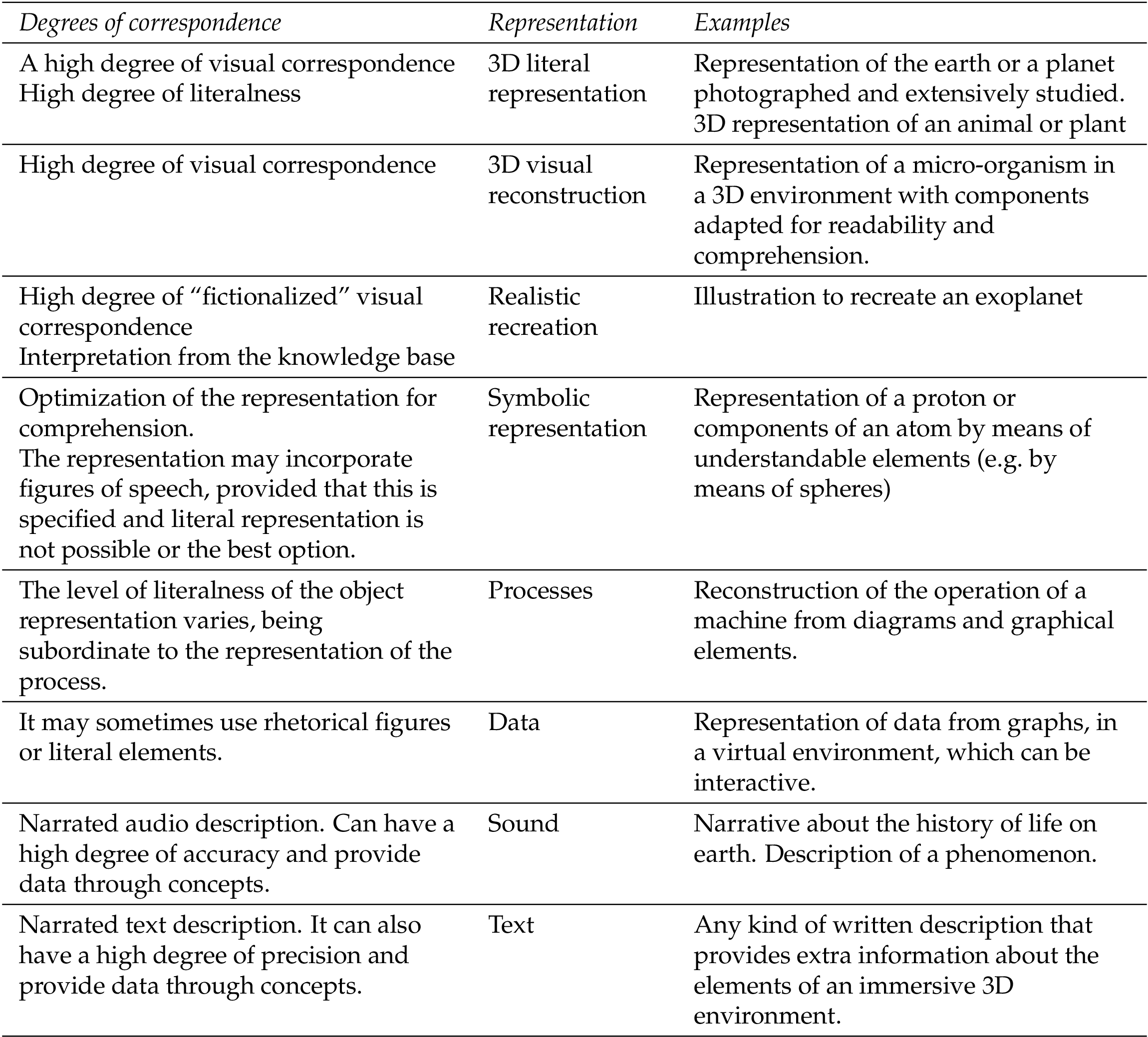
Additionally, rhetorical and communication fields propose scales like Villafañe’s Iconicity Scale [1985], which rates the likeness between an entity and its depiction across eleven levels, from natural imagery to abstract representation. This scale is crucial for determining the accuracy of information and its fidelity to the original subject.
Building on these principles, Table 5 is crafted to offer a taxonomical classification for representing information in scientific knowledge, specifically within XR and VR mediums. This taxonomy aims to facilitate information portrayal in XR and VR by harnessing their expressive capabilities and integrating narrative elements into 3D interactive environments. Given the complexity of XR and VR, this approach represents one facet of a broader model addressing various dimensions and methodologies necessary for effective representation in immersive technologies.
Exploring such similarities, it becomes possible to analyze and apply varying degrees of correspondence to other dimensions within the realm of scientific dissemination. In archaeology, for instance, the linear principle between “imagination” and “existence” in relation to the original maintains significant relevance in terms of representability. This progression can be observed from an abstract concept to a precisely replicated 3D object, preserving high resolution. Additional details regarding this progression are elaborated upon in Table 5, as part of framework elaborated by authors. It’s important to note that the table is adapted to the context of representation, considering that the factor of evidence is already explicitly formulated within scientific frameworks across different branches of knowledge.
The scales of evidence and iconicity are pivotal in constructing virtual worlds, focusing on the relationship between objects and their representations. The scale of evidence assesses the correspondence between an object’s original and remaining forms, while the scale of iconicity evaluates the likeness between an object and its depiction. In XR/VR, ensuring fidelity between the represented item and its original is crucial, especially for models depicting scientific concepts.
These scales serve as taxonomies to classify information across various research disciplines, emphasizing the optimal representation of information within a medium. They address how information is structured and adapted to fit the medium’s unique characteristics and the specific research field. In XR/VR, representation strategies should aid in classifying information types and integrating them into the narrative. By categorizing items based on their expression in the medium, developers and scientists can better design virtual environments to effectively convey scientific knowledge. XR/VR offers vast potential for knowledge representation, necessitating organized and systematic approaches to fully exploit the medium’s capabilities.
A proposed chromatic scale could visually represent the levels of correspondence and symbolism in scientific representations, enhancing communication of complex concepts. This suggestion builds on existing models like the archaeological scale of evidence, but with a focus on the object’s inherent properties. Such a chromatic scale, outlined in Figure 4 and Tables 5 and 6, would be part of an XR/VR Science Communication Model, offering a novel approach to categorizing and conveying scientific information within immersive environments.
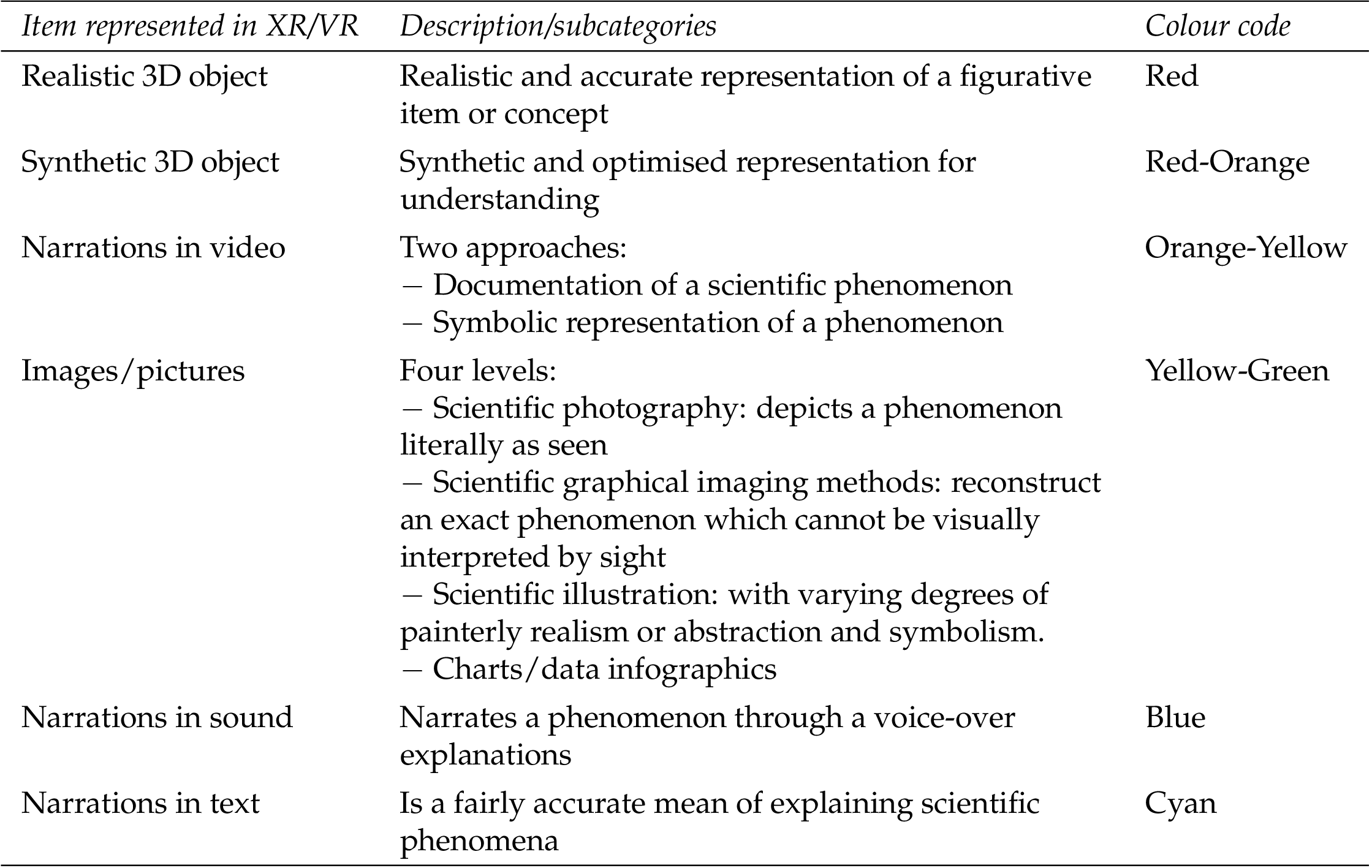
5.5 Narrative factors in the framework
Narrative and storytelling are crucial in designing content to communicate science effectively within virtual and extended realities. Integrating visual rhetoric and semiotics helps developers create environments that accurately represent scientific knowledge. Establishing principles to navigate the challenges of representing scientific concepts in immersive media is essential, with narrative serving as a key pillar.
Given the academic focus on narrative’s role in science representation, the development of models for XR and VR is critical. These mediums’ complexity, heightened by immersion and interactivity, underscores narrative’s significance in shaping user experience. Narrative elements like sound, images, characters, and interactive 3D objects contribute to a rich, multifaceted environment. In XR/VR, narrative transcends linearity, offering varying degrees of interactivity crucial for science communication. This shift necessitates guidelines to ensure that XR/VR maximizes scientific knowledge dissemination, leveraging the medium’s comprehensive potential.
Therefore, narrative encompasses various degrees of conceptualization and abstraction within the environment, treating components as narrative units. As depicted in Figure 5, these elements vary in their level of representation, design, structure, and narrative function. Understanding these variables is fundamental for scripting effective science communication in XR/VR, highlighting the importance of narrative in enhancing the representational fidelity and interactive quality of scientific content.
6 Discussion and projection
This study explored the feasibility of representing scientific knowledge within XR/VR. We noted the boundless potential of these technologies for information dissemination and XR/VR’s versatility in blending diverse media formats — from text and images to 3D objects. These features reveal the potential for XR/VR to communicate scientific content.
The developed “XR/VR Science Representation and Communication Model” framework, drawing from computer graphics, narrative, and human-computer interaction, aims to tackle the challenges of depicting complex scientific information in immersive environments. This model not only enhances the expressive power of XR/VR but also aids in cultivating a deeper comprehension of scientific concepts and fostering novel communication strategies suited to this immersive medium.
As XR/VR technologies progress, they promise enhanced interaction and narrative depth, highlighting the importance of ongoing research to refine information representation within these dynamic fields. The model presents a foundational framework for future exploration in the representation of scientific knowledge, positioning it as a crucial orientation point for subsequent studies.
Furthermore, this model illuminates the diverse elements of XR/VR, offering insights into the possibilities for representation within these platforms. It acts as an essential tool for deciphering the medium’s language rhetoric, facilitating the establishment of new communication protocols by leveraging XR’s interactive capabilities. Similar to the ways that literature, cinema, and photography have each developed unique codes and languages, XR/VR expands the expressive spectrum, accommodating a wide array of media and interactions.
This model also serves as a guide for structuring and categorizing integrated concepts within XR/VR, aiming to advance the development of standards that address narrative construction, language use, information depiction, and workflow optimization. It represents a multifaceted puzzle, each piece essential to the coherent assembly of scientific communication standards in immersive realities.
Acknowledging the rapid evolution of technological mediums, our model underscores the necessity for continuous research to enhance the portrayal of scientific knowledge in XR/VR. This ongoing endeavor is vital for maximizing the educational and communicative potential of virtual and extended realities.
Acknowledgments
This work was carried out with the support of the Spanish Ministry of Science and Innovation as part of the research project New interactive and immersive narratives to promote the Circular Economy and social innovation through scientific communication and citizen science from the School (eCOMCIENCIA) (PID2021-127019OB-I00).
7 Credit author statement
Jose Luis Rubio-Tamayo: Conceptualisation, Methodology, Validation, Formal Analysis, Investigation, Writing–original draft preparation, Writing–review and editing, Visualization, Supervision, Project Administration. Daniel Lewis Wuebben: Conceptualisation, Methodology, Validation, Writing–review and editing, Supervision. Manuel Gertrudix: Conceptualisation, Methodology, Validation, Writing–review and editing, Supervision, Project Administration, Funding Acquisition.
References
-
Allison, D. & Hodges, L. F. (2000). Virtual reality for education? In VRST ’00: Proceedings of the ACM symposium on Virtual Reality Software and Technology (pp. 160–165). doi:10.1145/502390.502420
-
Aparicio Resco, P. & Figueiredo, C. (2014). Escala de evidencia histórica — Scale of historical evidence. PAR — Arqueología y Patrimonio Virtual. Retrieved from https://parpatrimonioytecnologia.wordpress.com/2014/07/21/escala-de-evidencia-historica-scale-of-historical-evidence/
-
Aylett, R. & Louchart, S. (2003). Towards a narrative theory of virtual reality. Virtual Reality 7 (1), 2–9. doi:10.1007/s10055-003-0114-9
-
Al-Azawi, R., Albadi, A., Moghaddas, R. & Westlake, J. (2019). Exploring the potential of using augmented reality and virtual reality for STEM education. In L. Uden, D. Liberona, G. Sanchez & S. Rodríguez-González (Eds.), Learning technology for education challenges: 8th International Workshop, LTEC 2019, Zamora, Spain, July 15–18, 2019, Proceedings (pp. 36–44). doi:10.1007/978-3-030-20798-4_4
-
Azevich, A. I. (2019). Virtual reality: educational and methodological aspects. RUDN Journal of Informatization in Education 16 (4), 338–350. doi:10.22363/2312-8631-2019-16-4-338-350
-
Bailenson, J. N. & Yee, N. (2005). Digital chameleons: automatic assimilation of nonverbal gestures in immersive virtual environments. Psychological Science 16 (10), 814–819. doi:10.1111/j.1467-9280.2005.01619.x
-
Barnidge, M., Sherrill, L. A., Kim, B., Cooks, E., Deavours, D., Viehouser, M., … Zhang, J. (2022). The effects of virtual reality news on learning about climate change. Mass Communication and Society 25 (1), 1–24. doi:10.1080/15205436.2021.1925300
-
Biocca, F. & Levy, M. R. (1995). Virtual reality as a communication system. In F. Biocca & M. R. Levy (Eds.), Communication in the age of virtual reality (pp. 15–31). Hillsdale, NJ, U.S.A.: Lawrence Erlbaum. Retrieved from https://bit.ly/3XeiG1c
-
Bucher, J. (2017). Storytelling for virtual reality: methods and principles for crafting immersive narratives. doi:10.4324/9781315210308
-
Cáceres-Criado, I., García-Molina, D. F., Mesas-Carrascosa, F. J. & Triviño-Tarradas, P. (2023). New approach for optimizing the interpretation and representation of the degree of historical-archaeological evidence in the virtual reconstructions. Virtual Reality 27 (2), 967–983. doi:10.1007/s10055-022-00707-6
-
Chen, C. H., Yang, J. C., Shen, S. & Jeng, M. C. (2007). A desktop virtual reality Earth motion system in astronomy education. Educational Technology & Society 10 (3), 289–304. Retrieved from https://www.jstor.org/stable/jeductechsoci.10.3.289
-
Dede, C., Salzman, M. C. & Loftin, R. B. (1996). ScienceSpace: research on using virtual reality to enhance science education. In P. Carlson & F. Makedon (Eds.), Proceedings of World Conference on Educational Multimedia and Hypermedia & World Conference on Educational Telecommunications, 1996 (pp. 318–325). Boston, MA, U.S.A.: Association for the Advancement of Computing in Education (AACE). Retrieved from https://www.learntechlib.org/p/178602/
-
Denard, H. (2012). A new introduction to the London Charter. Retrieved from https://www.london-charter.org/introduction.html
-
Denard, H. (2013). Implementing best practice in cultural heritage visualisation: the London Charter. In C. Corsi, B. Slapšak & F. Vermeulen (Eds.), Good practice in archaeological diagnostics: non-invasive survey of complex archaeological sites (pp. 255–268). doi:10.1007/978-3-319-01784-6_15
-
Dincelli, E. & Yayla, A. (2022). Immersive virtual reality in the age of the Metaverse: a hybrid-narrative review based on the technology affordance perspective. The Journal of Strategic Information Systems 31 (2), 101717. doi:10.1016/j.jsis.2022.101717
-
Durukan, A., Artun, H. & Temur, A. (2020). Virtual reality in science education: a descriptive review. Journal of Science Learning 3 (3), 132–142. doi:10.17509/jsl.v3i3.21906
-
Finkler, W. & Leon, B. (2019). The power of storytelling and video: a visual rhetoric for science communication. JCOM 18 (05), A02. doi:10.22323/2.18050202
-
François, P., Leichman, J., Laroche, F. & Rubellin, F. (2021). Virtual reality as a versatile tool for research, dissemination and mediation in the humanities. Virtual Archaeology Review 12 (25), 1–15. doi:10.4995/var.2021.14880
-
Freina, L. & Ott, M. (2015). A literature review on immersive virtual reality in education: state of the art and perspectives. In I. Roceanu, F. Moldoveanu, S. Trausan-Matu, D. Barbieru, D. Beligan & A. Ionita (Eds.), Proceedings of the 11th International Scientific Conference “eLearning and Software for Education” (Vol. 1, pp. 133–141). doi:10.12753/2066-026x-15-020
-
Gallego, J. V. (1985). Introducción a la teoría de la imagen. Madrid, Spain: Ediciones Pirámide.
-
Green, S. J., Grorud-Colvert, K. & Mannix, H. (2018). Uniting science and stories: perspectives on the value of storytelling for communicating science. FACETS 3 (1), 164–173. doi:10.1139/facets-2016-0079
-
Harley, D., Tarun, A. P., Germinario, D. & Mazalek, A. (2017). Tangible VR: diegetic tangible objects for virtual reality narratives. In DIS ’17: Proceedings of the 2017 Conference on Designing Interactive Systems (pp. 1253–1263). doi:10.1145/3064663.3064680
-
Helbig, C., Bauer, H.-S., Rink, K., Wulfmeyer, V., Frank, M. & Kolditz, O. (2014). Concept and workflow for 3D visualization of atmospheric data in a virtual reality environment for analytical approaches. Environmental Earth Sciences 72 (10), 3767–3780. doi:10.1007/s12665-014-3136-6
-
Helsel, S. (1992). Virtual reality and education. Educational Technology 32 (5), 38–42. Retrieved from https://www.jstor.org/stable/44425644
-
Houck, L., Hassan, R., Thiis, T. K. & Solheim, K. (2013). Virtual Reality as a multidisciplinary communication tool. In P. J. Cruz (Ed.), Structures and architecture: new concepts, applications and challenges. doi:10.1201/b15267
-
Jackson, R. L., Taylor, W. & Winn, W. (1999). Peer collaboration and virtual environments: a preliminary investigation of multi-participant virtual reality applied in science education. In SAC ’99: Proceedings of the 1999 ACM Symposium on Applied Computing (pp. 121–125). doi:10.1145/298151.298219
-
Johnston, E., Olivas, G., Steele, P., Smith, C. & Bailey, L. (2018). Exploring pedagogical foundations of existing virtual reality educational applications: a content analysis study. Journal of Educational Technology Systems 46 (4), 414–439. doi:10.1177/0047239517745560
-
Joubert, M., Davis, L. & Metcalfe, J. (2019). Storytelling: the soul of science communication. JCOM 18 (05), E. doi:10.22323/2.18050501
-
Kavanagh, S., Luxton-Reilly, A., Wuensche, B. & Plimmer, B. (2017). A systematic review of virtual reality in education. Themes in Science and Technology Education 10 (2), 85–119. Retrieved from https://www.learntechlib.org/p/182115/
-
Lamb, R. L. & Etopio, E. (2019). Virtual reality simulations and writing: a neuroimaging study in science education. Journal of Science Education and Technology 28 (5), 542–552. doi:10.1007/s10956-019-09785-9
-
Liestøl, G. (2011). Situated simulations between virtual reality and mobile augmented reality: designing a narrative space. In B. Furht (Ed.), Handbook of augmented reality (pp. 309–319). doi:10.1007/978-1-4614-0064-6_14
-
Liou, W.-K. & Chang, C.-Y. (2018). Virtual reality classroom applied to science education. 2018 23rd International Scientific-Professional Conference on Information Technology (IT). doi:10.1109/SPIT.2018.8350861
-
Makransky, G., Petersen, G. B. & Klingenberg, S. (2020). Can an immersive virtual reality simulation increase students’ interest and career aspirations in science? British Journal of Educational Technology 51 (6), 2079–2097. doi:10.1111/bjet.12954
-
Manseur, R. (2005). Virtual reality in science and engineering education. Proceedings Frontiers in Education 35th Annual Conference. doi:10.1109/FIE.2005.1612051
-
Marini, D., Folgieri, R., Gadia, D. & Rizzi, A. (2012). Virtual reality as a communication process. Virtual Reality 16 (3), 233–241. doi:10.1007/s10055-011-0200-3
-
Markowitz, D. & Bailenson, J. (2019). Virtual reality and communication. Human Communication Research 34, 287–318. Retrieved from https://pdfs.semanticscholar.org/51be/85fb5d36c8e4927a252793b8a18cf9bd1eef.pdf
-
Martinez-Conde, S. & Macknik, S. L. (2017). Finding the plot in science storytelling in hopes of enhancing science communication. Proceedings of the National Academy of Sciences 114 (31), 8127–8129. doi:10.1073/pnas.1711790114
-
Matovu, H., Ungu, D. A. K., Won, M., Tsai, C.-C., Treagust, D. F., Mocerino, M. & Tasker, R. (2023). Immersive virtual reality for science learning: design, implementation, and evaluation. Studies in Science Education 59 (2), 205–244. doi:10.1080/03057267.2022.2082680
-
Mills, N., Courtney, M., Dede, C., Dressen, A. & Gant, R. (2020). Culture and vision in virtual reality narratives. Foreign Language Annals 53 (4), 733–760. doi:10.1111/flan.12494
-
Mintz, R., Litvak, S. & Yair, Y. (2001). 3D-virtual reality in science education: an implication for astronomy teaching. Journal of Computers in Mathematics and Science Teaching 20 (3), 293–305. Retrieved from https://www.learntechlib.org/p/9543/
-
Mori, M. (2012). The uncanny valley: the original essay by Masahiro Mori. IEEE Spectrum. Original article published in 1970. Retrieved from https://web.ics.purdue.edu/~drkelly/MoriTheUncannyValley1970.pdf
-
Ortiz-Cordero, R., León Pastor, E. & Hidalgo Fernández, R. E. (2018). Proposal for the improvement and modification in the scale of evidence for virtual reconstruction of the cultural heritage: a first approach in the mosque-cathedral and the fluvial landscape of Cordoba. Journal of Cultural Heritage 30, 10–15. doi:10.1016/j.culher.2017.10.006
-
Piovesan, S. D., Passerino, L. M. & Pereira, A. S. (2012). Virtual reality as a tool in the education. International Association for Development of the Information Society (IADIS) International Conference on Cognition and Exploratory Learning in Digital Age (CELDA). Madrid, Spain, Oct 19–21, 2012. Retrieved from https://eric.ed.gov/?id=ED542830
-
Riva, G. (1999). Virtual reality as communication tool: a sociocognitive analysis. Presence: Teleoperators and Virtual Environments 8 (4), 462–468. doi:10.1162/105474699566341
-
Rubio-Tamayo, J. L., Barrio, M. G. & García, F. G. (2017). Immersive environments and virtual reality: systematic review and advances in communication, interaction and simulation. Multimodal Technologies and Interaction 1 (4), 21. doi:10.3390/mti1040021
-
Ryan, M.-L. (2003). On defining narrative media. Image & Narrative 6. Retrieved from http://www.imageandnarrative.be/inarchive/mediumtheory/marielaureryan.htm
-
Ryan, M.-L. (2015). Narrative as virtual reality 2: revisiting immersion and interactivity in literature and electronic media. Baltimore, MD, U.S.A.: Johns Hopkins University Press. Retrieved from https://www.press.jhu.edu/books/title/11170/narrative-virtual-reality-2
-
Shim, K.-C., Park, J.-S., Kim, H.-S., Kim, J.-H., Park, Y.-C. & Ryu, H.-I. (2003). Application of virtual reality technology in biology education. Journal of Biological Education 37 (2), 71–74. doi:10.1080/00219266.2003.9655854
-
Shin, Y.-S. (2002). Virtual reality simulations in Web-based science education. Computer Applications in Engineering Education 10 (1), 18–25. doi:10.1002/cae.10014
-
Simões, R., Morais, C. & Moreira, L. (2019). Multimedia and virtual reality into communication practices of science centers: a social representations perspective. In ICERI2019 Proceedings. 12th annual International Conference of Education, Research and Innovation, Seville, Spain, 11–13 November, 2019 (pp. 4746–4752). doi:10.21125/iceri.2019.1165
-
Suzuki, W. A., Feliú-Mójer, M. I., Hasson, U., Yehuda, R. & Zarate, J. M. (2018). Dialogues: the science and power of storytelling. Journal of Neuroscience 38 (44), 9468–9470. doi:10.1523/JNEUROSCI.1942-18.2018
-
Taborda-Hernández, E., Rubio-Tamayo, J. L. & Rajas Fernández, M. (2022). Analysis of the narrative communication characteristics of virtual reality experiences: meaning-making components of the immersive story. Journal of Science and Technology of the Arts 14 (1), 9–31. doi:10.34632/jsta.2022.10055
-
Tham, J., Duin, A. H., Gee, L., Ernst, N., Abdelqader, B. & McGrath, M. (2018). Understanding virtual reality: presence, embodiment, and professional practice. IEEE Transactions on Professional Communication 61 (2), 178–195. doi:10.1109/TPC.2018.2804238
-
The London Charter for the computer-based visualization of cultural heritage (2006). Retrieved from https://www.london-charter.org/
-
The Seville Principles. International principles of virtual archaeology. (2017). Retrieved from http://sevilleprinciples.com/
-
Tibaldi, A., Bonali, F. L., Vitello, F., Delage, E., Nomikou, P., Antoniou, V., … Whitworth, M. (2020). Real world-based immersive Virtual Reality for research, teaching and communication in volcanology. Bulletin of Volcanology 82 (5), 38. doi:10.1007/s00445-020-01376-6
-
Vallance, M. & Towndrow, P. A. (2022). Perspective: narrative storyliving in virtual reality design. Frontiers in Virtual Reality 3, 779148. doi:10.3389/frvir.2022.779148
-
Weech, S., Kenny, S., Lenizky, M. & Barnett-Cowan, M. (2020). Narrative and gaming experience interact to affect presence and cybersickness in virtual reality. International Journal of Human-Computer Studies 138, 102398. doi:10.1016/j.ijhcs.2020.102398
-
Wickens, C. D. (1992). Virtual reality and education. In [Proceedings] 1992 IEEE International Conference on Systems, Man, and Cybernetics (Vol. 1, pp. 842–847). doi:10.1109/ICSMC.1992.271688
-
Wuebben, D., Rubio-Tamayo, J. L., Gertrudix Barrio, M. & Romero-Luis, J. (2023). 360° video for research communication and dissemination: a case study and guidelines. IEEE Transactions on Professional Communication 66 (1), 59–77. doi:10.1109/TPC.2022.3228022
Authors
Jose Luis Rubio Tamayo .
Lecturer at URJC. Researcher at Ciberimaginario Research Group. Bachelor’s degree in
Fine Arts (2007) and Ph.D. degree in Applied Creativiy (2015) from the Complutense
University of Madrid. He is currently a researcher in the Ciberimaginario Research Group,
and a lecturer in the Faculty of Communication at the University Rey Juan Carlos (URJC).
He has taught at other universities, such as the UNED (Spain), the UCM, and as a guest
lecturer at European universities such as the University of Porto (Portugal) and the
OAMK (Oulu, Finland). He has participated in several national and international research
projects related with topics such as communication and media, extended reality
technologies and science communication.
@JoseRubioTamayo E-mail: jose.rubio.tamayo@urjc.es
Daniel Wuebben
holds a Ph.D. degree in Literature from the Graduate Center of the City University of New
York. He is the author of Power-lined: Electricity, Landscape, and the American Mind (2019)
and is currently Assistant Professor of Teaching and Research in the Department of
Translation, Interpreting and Multilingual Communication and the Technology Research
Institute (IIT) at Comillas Pontifical University.
@WuebbenD E-mail: dlewis@comillas.edu
Manuel Gertrudix Barrio
received the Ph.D. in Science of Communication from the University Complutense de
Madrid, Spain, in 1999. He is currently Associate Professor at URJC and Coordinator
of Ciberimaginario Research Group. He has coordinated several national and
international research projects in the domain of scientific communication and citizen
science. He has published more than 50 research articles and book chapters, among
others.
@gertrudix E-mail: manuel.gertrudix@urjc.es